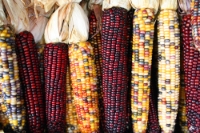
In the last fifty years, global agricultural practices have favored growing ever fewer varieties of high-yielding crops, leading to fears that the loss of genetic diversity in food leaves the growing human population exposed to risks of food shortages from disease, pests, and climate change.
With the ongoing East African drought crisis, the persisting threat of global climate change, and the world population now estimated at 7 billion, global concerns about food insecurity are again in the news. Little mentioned, however, is the continuing loss of genetic diversity of the foods we eat today—a trend that has rapidly accelerated since the twentieth century and that raises troubling questions about the vulnerability of the world's food supply. One attempt to maintain plant biodiversity has been the establishment of gene banks—giant vaults to store seeds collected from around the globe. But there are serious questions over whether the collection of seeds from ancient Mesopotamian wheat, South American potatoes, or tropical plants in an isolated arctic catacomb can undo a recent history of agriculture that has emphasized bigger yields through modern, standardized varieties of crops.
Readers may also want to see these Origins articles on the Global Food Crisis; Population Growth in India and the World Water Crisis
Historians still squabble over whether there really was a "first" American Thanksgiving. But a handful of documents give us a hint at what might have been served: likely roasted venison and fowl—probably turkey and a number of other wild birds—dried Indian corn, wheat, barley, and fish. The local diet also included lobster, eel, nuts, squash, beans, and berries.
Today's Thanksgiving feast similarly celebrates the bounty of nature, though many of the varieties of corn, squash, and other fruits and vegetables Native Americans and European settlers farmed no longer exist.
Four centuries later, we have come to depend increasingly on only a handful of commercial plant varieties for our food supply. And we see signs everywhere of what some observers call the sinking ark of agricultural biodiversity (agrobiodiversity).
As we sit at the table to give thanks, most of us eat the same commercial variety of turkey—the Broad-breasted White (BBW)—fed with genetically modified corn and soy meal in giant turkey mills. Our stuffing is made from a handful of wheat, corn, and soy varieties cultivated with tractors and fertilizers and bred to resist pests, plagues, and drought. And when you pass the potatoes, you're probably passing one of the three kinds that, since the 1970s, have made up three-quarters of the U.S. potato crop.
In the United States, of 7,000 apple varieties that were grown by the 1800s, fewer than a hundred are cultivated today. More than nine out of ten of the varieties in the official U.S. Department of Agriculture seed list of 1903 were no longer available by the 1980s.
This genetic erosion is common throughout the planet as a result of changing agricultural practices. Wherever we look, we see the rise in uniformity of agricultural plant varieties and a loss of genetic diversity, with many traditional varieties and wild relatives of today's crops simply disappearing.
Over the millennia that humans have engaged with agriculture, about 7,000 plant species have been cultivated or collected for food. But today, according to the United Nations' Food and Agriculture Organization (FAO), fewer than 150 species are under commercial cultivation and only 30 species provide 95 percent of human food energy needs. In fact, just four of them—rice, wheat, maize and potatoes—provide more than 60 percent of human dietary energy supply.
The narrowing of crop diversity has accelerated to frightening proportions in recent decades as a result of three processes: the introduction of commercial, scientifically hybridized plant varieties (mainly since the mid-twentieth century); the expanded use of certain high-yielding varieties as part of the Green Revolution in agricultural production of the 1960s and 1970s; and the expansion of industrial agriculture.
Growing genetic uniformity poses a variety of possible threats to the human food supply. As awareness of the problem has grown over the past three decades, governments, international organizations, and businesses across the world have begun to store available genetic material in gene banks—vaults where scientists conserve seeds away from their original habitats in specially designed buildings at temperatures below freezing.
But are these seed arks enough to stave off a potential food catastrophe? And what other ways are there to ensure human food security? In the long term, keeping farmers on the farm cultivating a wide diversity of locally adapted crops may be the best solution.
The Perils of Declining Food Crop Diversity
A number of risks accompany the loss of genetic diversity in agriculture, including crop disease, pests, climate change, and the rising human population.
Cultivating large areas with one or two high-yielding crop varieties can be disastrous when that crop falls victim to disease. To take one recent example: In Brazil, the world's largest producer and exporter of oranges and orange juice, the genetic uniformity of the country's sweet orange trees has left the citrus industry susceptible (since 1987) to a bacterial disease that causes economic losses that were as high as $250 million U.S. dollars in 2000.
The most famous case of the disastrous outcomes of monoculture is probably the Irish potato famine. European colonizers introduced the potato to European cuisine, and it became the main staple crop in the cold, rainy climate of Ireland. Irish farmers planted primarily one potato variety, the Lumper potato, which was exposed to a deadly fungus in 1845.
Because of genetic uniformity, the fungus contaminated and wiped out much of the potato crop. In the following decade, the famine killed approximately one million people and resulted in the emigration of another million from Ireland.
These days, a major new risk is at the door. A virulent cereal stem rust (Ug99) now attacks previously resistant genes worldwide. The fast mutating fungus, first identified in Uganda in 1999, has now spread across Sub-Saharan Africa, North Africa, and the Middle East. Scientists predict that Ug99 will infect other areas, including North America, in less than ten years.
Because of the spread of monocultures and the narrowing of wheat's genetic basis, almost 90 percent of the world's wheat is defenseless against Ug99. Not only local farmers but also commercial breeders and scientists have to find and develop adaptive traits, which is only possible when we have agrobiodiversity.
Agrobiodiversity conserves multiple food species, ensures genetic variability within species, and preserves diverse farming techniques and knowledge. It allows farmers to switch quickly from one crop variety to another when a certain strain no longer produces good results in the local environment.
In the Peruvian Andes (in contrast to Ireland), where potatoes were first domesticated about 13,000 years ago, Incas cultivated several potato varieties as insurance against crop failures. Today, Andean farmers still cultivate multiple potato types in different shapes, colors, and flavors for reasons of culture, diet, and food security.
Heterogeneous genetic characteristics provide several benefits such as agronomic qualities like resistance to pests, diseases and drought, and adaptations to abiotic stresses such as salinity tolerance.
A Turkish wheat landrace collected in 1948 was found to carry genes for resistance and tolerance to various disease causing fungi. Plant breeders in the United States have used these genes to breed wheat varieties that are resistant to a range of diseases. These genes became a parent of many of the wheat cultivars now grown in the northwestern United States.
A dwarf wheat landrace from Japan was introduced to the United States in 1946. It was used as a donor of dwarfing genes, which increased production by improving nitrogen uptake. Similarly, Zerazera sorghums from Ethiopia have provided resistance to downy mildew for many inbred lines in the U.S. and Mexico.
Agrobiodiversity also provides a foundation for food security, livelihoods, and insurance by sustaining agriculture in the face of global environmental threats such as climate change. Climate change could, for example, make it impossible to grow a certain crop over a large area where it is now cultivated. Meanwhile other plant varieties that might have flourished under the changed conditions have been lost to monoculture itself. The absence of crop diversity certainly renders humanity less adaptable to changes in climate.
Of course, the rapidly growing human population makes food security an ever more acute problem. With the world's population recently passing seven billion—and with a larger portion of those humans now demanding a diet rich in meat—a restriction in the global food supply would mean a human catastrophe. [Read Origins for more on food systems and rising population.]
Rising Malthusian fears that agriculture cannot keep up with a rapidly expanding population have worsened with the threat of climate change and new diseases. Policymakers have been slow to address the threats posed by the genetic erosion of agriculture, often demanding more evidence and questioning its implications for food security.
The issue before us is how to use, conserve, and sustain agrobiodiversity, while we depend increasingly on a limited number of commercial varieties for our food supply.
A Brief History of Seeds and Hybrids
First as gatherers, then as farmers, humans have long relied on plants for food security and self-sufficiency.
Agriculture represents a critical interaction of people and nature. For millennia, farmers have carefully selected and bred new crop varieties from semi-wild relatives of crops in order to optimize their yields in local conditions.
Food security in many parts of the world still depends on the availability of such locally adapted crops. Farmers in isolated areas of Turkey, for example, still cultivate the semi-wild relatives of wheat cultivated 8,000 years ago. Corn (maize) biodiversity in Mexico still endures despite pressures on farmers for modernization.
At the same time, humans have long moved genetic resources (in the form of seeds) from one place to another through migration and trade.
Agricultural crop seeds were exchanged on the Silk Road. In the so-called Columbian Exchange that followed in the wake of Columbus's "discovery" of the Americas, European colonizers transformed agriculture both in the colonies and in Europe by bringing seeds, animals, germs, and other goods from one continent to the other.
Yet, for all humanity's long history with agriculture, the twentieth century witnessed the rapid creation of hybrid seed varieties and modified crops, which quickly spread across the globe. Seed manipulators are no longer farmers experimenting with different strains and species on their farms, but scientists employed by agribusiness to produce new genetic varieties in their laboratories.
With the development of Mendelian genetics in the nineteenth century and the rise of a seed industry as part of modern agriculture, seed breeding has become both a scientific and commercial activity.
In the United States, hybrid corn (maize) was produced as early as 1909. By the mid-1920s, public agricultural research institutions and land-grant colleges were training farmers to produce their own hybrid seeds. The development of hybrids enabled an increase in farm output, but also allowed breeders to assert control over the seed supply.
The U.S. Plant Patent Act of 1930 enabled patents of new plant varieties, increasing the commercial impetus for companies to gain control over seeds and to tackle pressing agricultural dilemmas.
For example, one of the oldest adversaries of wheat and barley is stem rust, caused by a fungus that affected crop cultivations for centuries. It contributed to major crop losses in the United States in the 1930s and 1950s.
In the 1950s, a number of stem rust-resistant genes were described, cataloged, and collected from several wheat varieties from Europe and the Middle East to create new resistant wheat varieties in the United States. These were then cultivated widely, from Africa to Europe, and from China to the United States. The incidence of stem rust was reduced almost to insignificance by the mid-1990s.
Between 1940 and the 1950s, as seed companies stepped into the crop hybridization process—and produced new, high-yield, resistant varieties—their profits tripled.
Technology transformed agricultural research and development. Seeds became not an outcome of farmers' ingenuity but a private commodity. New varieties of seeds could no longer be saved or traded, but had to be purchased by farmers.
Protection of the scientific knowledge of modern seed production promoted further innovation in seed science, but mainly protected the rights of private companies—sidestepping farmers in the seed development process.
Thus, "progress" in agriculture has often been premised on a distinction between "modern" crop varieties, including high-yielding, certified, and now genetically engineered crops, and farmer-saved or semi-wild relatives of crops also known as traditional varieties.
These modern crop varieties reflect the mentality of recent agriculture that lionizes a technological-fix approach. It relies on a simple assumption: If we increase food supply through increased yields, we will address hunger and food security.
This narrow focus on "progress" through modern varieties has facilitated the further loss of diversity on the farm and at the dinner table.
The Green Revolution
With the belief that these new, modern, commercially protected varieties and techniques were the way of the future, it only made sense for the businesses that produced them that they should export their products to the rest of the world.
In the postwar period, new hybrid varieties were introduced in population-dense countries, such as India and Pakistan, leading to the doubling of wheat yields and food production and probably saving a billion people from hunger. This international effort, now called the Green Revolution, helped expand modern industrialized agriculture into the developing world.
The Green Revolution was comprised of a particular package of land-saving technologies and practices that required high-yielding grain varieties (especially wheat and rice bred at international research centers), more chemical fertilizers, and extensive irrigation. This package was in turn supported with state subsidies, credit, and price controls.
The Green Revolution reflected prevailing Western ideas about the modernization of agriculture. Its proponents applied scientific principles to agricultural processes to improve yields in developing countries in an attempt to escape perceived Malthusian limits on food supply.
High-yielding varieties were seen as the future of agriculture, whereas traditional varieties were considered remnants of past eras of prolonged scarcity.
Foreign experts collaborated with local farmers to teach new agricultural techniques. Officials from the U.S. Agency for International Development (USAID) and experts from other international organizations carried out thousands of meetings in villages across the so-called developing world to teach modern agriculture to farmers.
State officials throughout the globe embraced modern plant varieties and agricultural modernization policies as progress. In the process, they frequently excluded traditional varieties (often well adapted to local conditions) from national agricultural policies.
But increased agricultural yields came with a cost. The new varieties required increased chemical and water use. In developing nations, often only the richer farmers could afford to pay for the seeds, agricultural chemicals, and irrigation required to sustain high yields. Many smaller farmers were driven out of business.
Globally, farmers relinquished seeds that had been cultivated over generations. In Turkey, a center of agricultural domestication and diversity of wheat, the Green Revolution led to the replacement of hundreds of local wheat varieties with high-yielding dwarf wheat varieties introduced from Mexico. In the 1970s, Turkey also imported and started to cultivate high-yielding varieties improved from American and Russian wheat cultivars.
Rice farmers in the Philippines, similarly, gave up the Taiwanese variety they had long planted. They first sowed one hybrid rice variety, but it was attacked by disease. Another hybrid variety proved resistant to disease, but susceptible to strong winds. When the farmers wanted to return to their original Taiwanese variety, they found out there were no more farmers in their communities or in Taiwan who cultivated it.
The Green Revolution's legacy is fraught with disagreement.
Norman Borlaug, the father of the Revolution, asserts that the movement set out to alleviate hunger and succeeded: The world was able to produce an additional 1.9 billion tons of grains, an over 170 percent increase, from the 1950s through the 1990s on the same amount of land. Mass hunger would have ensued without these changes.
However, not everyone has been so sanguine about the results. Vandana Shiva, a physicist and environmental activist, argues that the Green Revolution prolonged poverty and brought dependence on Western technology to the developing world. She argues the main beneficiaries were the agrochemical industry, large petrochemical companies, and manufacturers of agricultural machinery in the West.
Whether the Green Revolution made life better or worse for the growing populations of the developing world, it certainly encouraged seed sales, which mostly benefitted companies from wealthier, developed countries. Many farmers must now buy seeds that are patented and protected under laws and agreements protecting breeders' rights. Some are derived from the very seeds they once gave and received for free.
International agreements, such as the 1961 International Convention for the Protection of New Varieties of Plants, protected private companies by giving breeders exclusive control over new varieties. Last revised in 1991, the Convention accommodates capital intensive, large-scale commercial agricultural systems.
The World Trade Organization and its Trade Related Intellectual Property Rights (1994) agreement have further established a uniform legal and policy infrastructure for intellectual property rights in each member country. Although countries can implement their own system of plant protection under these regulations, there is a narrow focus across the globe on more commercialization and privatization of plant genetic material used for agriculture and food.
For their part, many developing countries have also introduced new seed regulations that limit farmers' right to exchange, save, and store seeds from their farms on a national scale.
Technologies such as satellite images and plant fingerprinting have enhanced the ability to monitor intellectual property right infringements, greatly reducing farmers' access to seed resources.
Seed Banks as the Great Human Insurance Policy?
One response to rapidly dwindling agrobiodiversity has been to gather and safely store seeds of crop varieties in controlled environments. Gene banks or seed banks are located away from farms where the seeds are cultivated and serve as safety deposit boxes.
Other similar off-site conservation mechanisms include botanical gardens, DNA libraries, greenhouses, and other endeavors by agricultural research institutions.
The Soviet Union was the first to establish gene banks for crops. However, Russian botanist Nikolay Vavilov's effort to collect seeds worldwide in the 1920s and 1930s was aimed at research alone, not the protection of seed diversity.
The United States started germplasm collection in the late 1940s and established its first gene bank in 1959. Unlike in the Soviet Union, these resources were used for agricultural production.
At the international level, the idea of a network of gene banks gained traction following the 1970 outbreak of the corn leaf blight in United States. New global partnerships, such as the Consultative Group on Agricultural Research (CGIAR), began to establish international agricultural research centers beginning in the 1970s.
Working in collaboration with hundreds of governments, civil society organizations, and private businesses around the world, CGIAR today supports 15 international centers for agricultural research and about 1,750 gene banks. Together, these gene banks contain a total of 6 million accessions of all crops and represent 95 percent of all cereal landraces worldwide. These are public or non-profit entities whose goal is to sustain "food for people."
The CGIAR gene banks are located primarily in the global South but their funding and guidance comes primarily from Northern donors. CGIAR ensures that seeds and plant germplasm are stored in duplicate and kept below freezing so that they can remain viable for decades. They are cultivated under sterile conditions with fertilizers.
CGIAR centers are open access institutions: The accessions cannot be patented, and they are distributed free upon request to all their member states. Countries submit their genetic resources on a voluntary basis. Yet, 45 percent of global gene bank collections are held in just seven countries, a concentration of resources that raises questions about the need for facilitated global access.
Many countries continue to depend on CGIAR's gene banks to improve their agriculture, taking advantage of the CGIAR's open access to resources for research, breeding, conservation, and training. Between 1974 and 2001, Kenya and Uganda received a total of 12,000 unique accessions from all CGIAR gene banks that were collected from other countries. In the same period, about 4,000 accessions collected from Kenya and Uganda were distributed to the world.
Seeds and Climate Change
There is now an increased interest in global seed collection and storage because of the threat of climate change.
The most ambitious is the Svalbard Global Seed Vault, established in 2008 and nicknamed "the doomsday vault," which sits inside the permafrost of a sandstone mountain on a Norwegian island just a few hundred miles from the North Pole. It professes to be a backup for global collections already stored in CGIAR centers. It is located in a permanently chilled, earthquake free zone, some 400 feet above sea level to ensure that the seeds will be viable when climate change shifts landscapes and agricultural zones.
Since 2000, the Millennium Seed Bank Project of the Kew Royal Botanical Gardens in the United Kingdom has also collected and banked over a billion seeds worldwide from 24,000 different plant species. The goal of the project is to collect and save 25 percent of the world's dryland wild plants by 2020.
A similar recent endeavor is Project Baseline in the United States. Supported by the National Science Foundation, the project will enable the collection of 12 million seeds in the next four years and will act like a "time capsule" for evolutionary biologists against climate change threat.
Seed Banks and their Discontents
Crop scientists and human ecologists often suggest that gene banks alone cannot conserve seeds because the genetic diversity of crops develops differently on the farm than when conserved off-site in gene banks.
Indeed, international agreements, including the international Convention on Biological Diversity—a global agreement for the conservation of biological diversity signed at the Rio Earth Summit (1992)—stress not only the importance of agrobiodiversity and the conservation of seeds but the conservation of seeds on farms and by farmers in order to guarantee long-term food security.
Agrobiodiversity is a result of the interaction between the crop and local human population, and freezing genetic material in gene banks may stop the clock: Crops cannot continue to transform genetically in response to human decisions and environmental changes.
Gene banks or seed banks may also be susceptible to equipment failure, attacks, or—perhaps most importantly—financial problems, since they are costly to run.
There is also a question of access. Whereas many of the CGIAR centers are open access resources, the newer ones are not. Both the Svalbard and the Millennium Seed Bank are more restrictive, with access limited to those with permission from countries that make deposits.
A further concern is that gene banks conserve only seeds or genetic material, but not necessarily the traditional knowledge associated with those seeds. Information about the location where the seed was collected provides only limited knowledge about why and how farmers have bred and continue to cultivate that particular variety.
Moreover, collections by seed or gene banks are selective and cannot represent all of the seed varietals that have been cultivated by farmers worldwide. When a new high-yielding variety becomes available—such as through the Green Revolution, genetic modification technology, or other means—pressure for the extinction of the existing traditional varieties grows—as happened to rice farmers in the Philippines.
Seeds, Farmers, and Traditional Knowledge
In the long run, the most efficient way to conserve agrobiodiversity is to maintain farmers' cultivation of traditional varieties.
On-the-farm conservation by farmers incorporates indigenous knowledge, crop-pest co-evolution, and security through redundancy and decentralization.
A wheat farmer may grow different wheat varieties to be used as animal feed, for markets, or for household consumption. The farmer may consider ecological niches: The wheat variety suitable for hillside may not be appropriate for land at the valley bottom. The farmer may choose different varieties for particular strengths, such as resistance to pests, or simply to enjoy the taste for bread.
Farmers rely on diversity on the farm and in their communities. When one crop fails or seeds no longer provide enough yield, farmers can plant other varieties since they have access to other seeds. Farmers also renew seeds, if the seeds no longer meet their expectations of yield, taste, or sales at the market.
On-the-farm conservation serves as a continuous source of genetic material for gene bank-based conservation and gives countries with traditional crop varieties the option of promoting conservation with wider human participation. It also recognizes the role and contribution of farmers to agriculture, and food security for the whole world. Of course, maintaining seeds on the farm also helps maintain farmers' present and future livelihoods.
Today, food security in many parts of the world—especially in impoverished countries—depends on crop genetic diversity especially in the form of agrobiodiversity cultivated by farmers in their fields. But the Green Revolution and the spread of industrial agriculture more broadly has led to the genetic uniformity of crops worldwide.
We now have the same wheat varieties from Mexico to Turkey and from Kenya to India, with the same genetic material, that produce maximum yields but also leave us susceptible to agricultural collapse from disease, pests, changing climate, and rising population.
History warns us of the dangers of putting all our wheat—hybrid or otherwise—in one basket.
Brush Stephen, ed. Genes in the Field: On-farm Conservation of Crop Diversity. Washington D.C.:Lewis Publishers, Ottowa: IDRC, and Rome: IPGRI, 2000.
Food and Agriculture Organization. The State of the World’s Plant Genetic Resources for Food and Agriculture (First Report), 1996.
Heywood, V.H., ed. Global Biodiversity Assessment. United Nations Environment Programme. Cambridge: Cambridge University Press, 1995.
Juma, Calestous. The Gene Hunters: Biotechnology and the Scramble for Seeds. London: Zed Press, 1989.
Kloppenburg, Jack. First the Seed: The Political Economy of Plant Biotechnology 1492-2000. (2nd ed).The University of Wisconsin Press, Madison. 2004.
Tansey, Geoff and Tasmin Rajotte, eds. The Future Control of Food: A Guide to International Negotiations and Rules on Intellectual Property, Biodiversity and Food Security. London: Earthscan, 2008.
Useful Links
Food and Agriculture Organization of the United Nations
Botanic Gardens in Conservation, The Millennium Seed Bank Project
The Global Crop Diversity Trust, The Svalbard Seed Vault.
The International Treaty on Plant Genetic Resources for Food and Agriculture